Scaling chemical etching to high volumes isn’t just about bigger tanks—it’s a holistic strategy encompassing throughput optimization, cost control, smart automation, proven case methodologies, and robust risk management. By applying these tactics, operations directors and supply chain planners can transform etching from a niche process into a high‑capacity, world‑class manufacturing pillar—delivering complex metal parts at scale, on time, and on budget.
Throughput Optimization: Batch Processing vs. Conveyorized Systems
Maximizing parts-per-hour (PPH) hinges on choosing the right equipment and workflow:
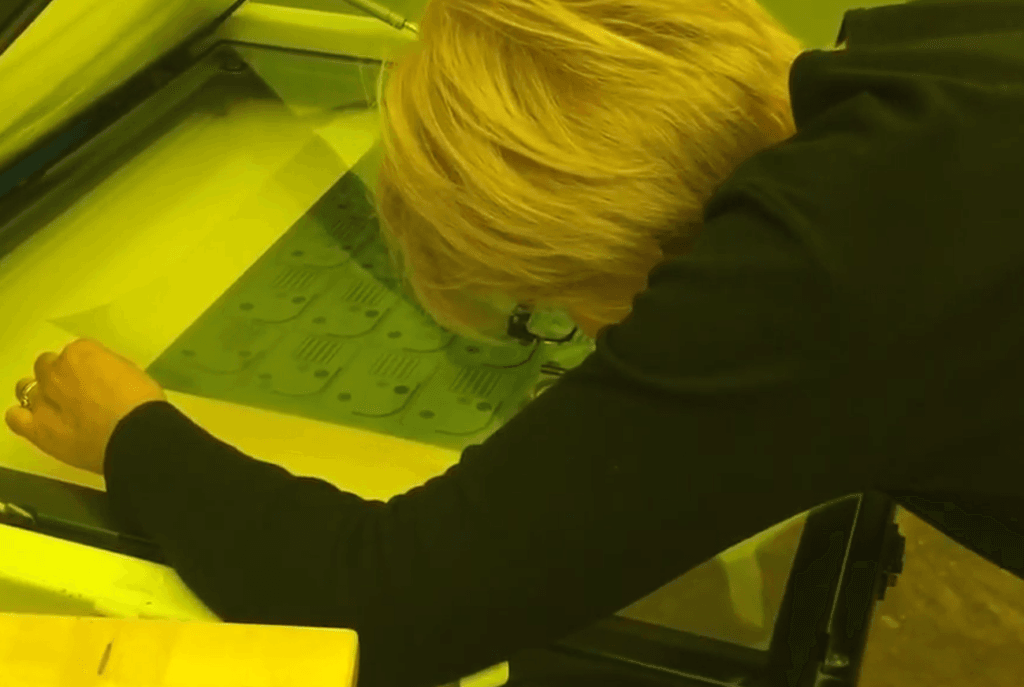
System Type | Capacity (PPH) | Typical Batch Size | Flexibility |
---|---|---|---|
Batch Processing | 500–1,000 | 10–500 parts | High flexibility for varied part sizes |
Conveyorized Systems | 5,000–20,000 | Continuous flow | Ideal for stable, long‑run jobs |
- Batch lines excel when you need frequent changeovers or multiple alloys in short runs.
- Conveyorized etch lines, like Masteretch’s 750 Series (capable of 30″‑wide conveyors and modular throughput scaling), drive continuous, high‑volume output without manual handling delays (Masteretch Chemical Etching Equipment).
By mapping your demand profile against these capacities, you can right‑size investments and avoid bottlenecks in your production flow.
Cost Drivers: Raw Material Utilization & Etchant Replenishment
Controlling cost per part requires vigilance over both metal yield and chemical consumption:
- Raw Material Utilization: Chemical etching routinely achieves 90% sheet‑utilization rates—far exceeding the 50% typical of CNC milling—directly reducing scrap costs.
- Etchant Replenishment: Establish a scheduled top‑off frequency based on bath volume and throughput. For example, a 1,000‑liter ferric chloride bath handling 10,000 parts/week may require a 10% replenishment every 2 weeks to maintain consistent etch rates and surface quality.
Optimizing these drivers can cut overall manufacturing costs by up to 30%, according to industry benchmarks.
Automation Integration: AI‑Powered Vision & Robotics
Scaling to millions of parts demands automation that maintains quality without slowing throughput:
- Vision‑Based Defect Detection: AI‑powered systems (e.g., Cognex In‑Sight® vision sensors) detect etch defects—pinholes, over‑etching, mask lifts—with 99.98% accuracy, flagging issues before rinse cycles.
- Robotic Part Handling: Integrating collaborative robots for loading/unloading minimizes manual errors and supports 24/7 operation, boosting effective uptime to >95%.
Adopting these technologies turns your etch line into a self‑optimizing production cell, reducing labor costs and ensuring consistent, repeatable quality.
Case Study: Automotive Supplier Etching 5 Million+ EV Battery Contacts Annually
Precision Micro, a leader in photochemical etching, scaled their EV battery contact production to over 5 million units per year, achieving astonishingly low defect rates:
- <0.1 PPM Defects: Through a combination of rigorous process control, closed‑loop etchant regeneration, and AI inspection, the supplier maintained industry‑leading quality.
- Lean Supply Chain: By consolidating mask preparation, etch, and finish plating into a unified line, they eliminated intermediate handling and cut lead times by 60% (Precision Micro EV Etching Insights).
This success story underscores how end‑to‑end integration can drive both volume and excellence in high‑stakes automotive applications.
Risk Mitigation: Dual‑Source Suppliers & ISO 14001 Compliance
Ensuring uninterrupted operations and environmental stewardship is paramount:
- Dual‑Source Chemicals: Partner with at least two ferric chloride and ammonium persulfate suppliers to shield against raw‑material shortages or price spikes.
- ISO 14001 Environmental Management: Certification not only demonstrates your commitment to sustainable waste management but also streamlines permitting and regulatory approvals (ISO 14001 Overview).
By embedding these safeguards, you protect throughput—and your bottom line—against supply‑chain and compliance risks.